Role of Vitamins ability to acquire energy from Fats.
- James Shmagranoff
- Jun 6, 2019
- 4 min read
Both water and fat soluble vitamins play an integral role in a variety of metabolic processes through the macronutrients protein, carbohydrates, and fats. With regards to lipids, beta oxidation is highly affected by consumption of and circulating levels of the water soluble vitamins Riboflavin, Niacin, Pantothenic Acid, Pyridoxine, Biotin, Ascorbic Acid and the fat soluble vitamins A and D. Beta-Oxidation, which primarily takes place inside the mitochondria of cells and via similar pathways in peroxisomes is a multistep chemical process in which fatty acids are broken down and yield energy (Garrett & Grisham, 2013). As mentioned earlier, vitamins play a variety of roles within the body related to the conversion of food into energy.
Vitamin B2 also known as riboflavin is essential to normal functioning enzyme activity within the body. Riboflavin functions as a source of FMN (flavin mononucleotides) within the body which play a role in REDOX reactions (Powers, 2003). FMN is utilized by flavoproteins in REDOX reactions including the oxidation of fatty acids, REDOX reactions are simply the transference of electrons. Niacin, also a B vitamin, more specifically known as B3, also plays a role in lipid oxidation but via negative effects. Research on supplementation of doses in the 2g per day range noted decreases in fat oxidation rates over a two week duration period while supplementing with Niacin at this dose (Kelly, Lawson, Campbell, Storlien, Jenkins, Whitworth, & O’Sullivan, 2000). Niacin can therefore have a suppressive effect on lipolysis when consumed in singular higher doses (Kelley et al., 2000).
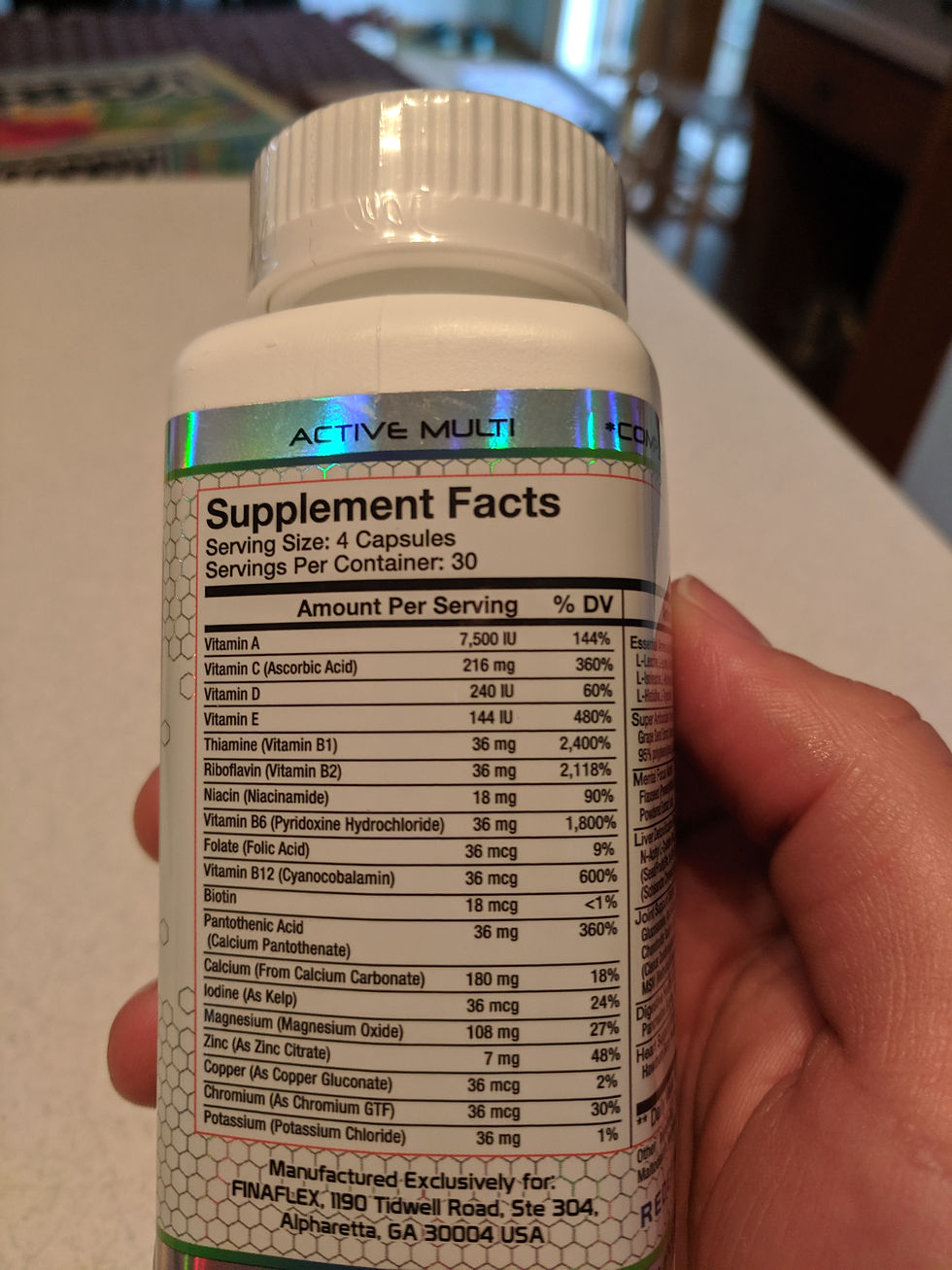
Panthothenic Acid, Vitamin B5, is considered rate limiting in oxidation levels of fat during exercise, as it is required in the productions of Coenzyme A (Sahlin, Sallstedt, Bishop, & Tonkonogi, 2008). A function of coenzyme A is to bind the thiol ester of the fatty acid to its thiol groups to begin beta oxidation (Garrett & Grisham, 2013). Biotin is stored in acetyl Co A Carboxylase (Tong & Harwood, 2006). This specific enzyme is a catalyst and functions to inhibit the conversion of acetyl CoA into malonyl CoA, which acts an inhibitor of fatty acid oxidation (Tong & Harwood, 2006). This makes the water soluble vitamin biotin the rate limiting factor. Vitamin C, as discussed in the week 1 discussion post, affects the synthesis of the amino acid derived canitine, which acts as a transporter of fatty acids to the mitochondria of cells (Wutzke & Lorenz, 2004). Without sufficient levels of vitamin C present, 4-butyrobetaine levels rise, inhibiting carnitine levels and, thus, fatty oxidation levels in the body (Johnston, Solomon, & Corte, 2013).
Sub optimal intake of vitamin C has been linked to alterations in plasma free carnitine levels (Johnston, Solomon, & Corte, 2013). In the presence of low vitamin C intake, 4-butyrobetaine hydroxylase is decreased and 4-butyrobetaine levels rise, which acts as a potent inhibitor of carnitine transport in skeletal muscle (Johnston, Solomon, & Corte, 2013). Fat soluble vitamin A also plays a functional role in lipid metabolism, with pathways being linked to expression of lipogenic genes in the liver (Zhao, Li, Li, Chen, Zhang, & Chen, 2012). Vitamin D causes an up regulation of lipid oxidation due to activating genes involved in mitochondrial metabolism (Marcotorchino, Tourniaire, Astier, Karkeni, Canault, Amiot, Bendahan, Bernard, Martin, Giannesini, Landrier, 2014).
In conclusion, there is a number of water soluble and fat soluble vitamins utilized in beta oxidation of fat. From enzyme activities to rate limiting factors, the importance of vitamins in metabolic processes cannot be stressed enough.
Sources
Garrett, R. H., & Grisham, C.M. (2013). Chapter 23: Fatty acid catabolism. Biochemisty (5th ed.)(pp.761-785). Belmond, CA: Brooks/Cole Cengage Learning
Johnston, C.S., Solomon, R.E., & Corte, C. (2013). Vitamin C depletion is associated with alterations in blood histamine and plasma free carnitine in adults. Journal of the American College of Nutrition, 15(6), 586-591. Doi:10.1080/07315724.1996.10718634
Kelly, J.J., Lawson, J.A., Campbell, L.V., Storlien, L.H., Jenkins, A.B., Whitworth, J.A., O’Sullivan, A.J. (2000). Effects of nicotinic acid on insulin sensitivity and blood pressure in healthy subjects [abstract]. Journal of Human Hypertensions, 14(9), 567-72. Retrieved from https://www.ncbi.nlm.nih.gov/pubmed/10980588
Marcotorchino, J., Tourniaire, F., Astier, J., Karkeni, E., Canault, M., Amiot, M.J., Bendahan, D., Bernard, M., Martin J.C., Giannesini, B., & Landrier, J.F. (2014). Vitamin D protects against diet-induced obesity by enhancing fatty acid oxidation. Journal of Nutrition Biochemistry, 25(10), 1077-1083. doi: 10.1016/j.jnutbio.2014.05.010
Powers, H.J. (2003). Riboflavin (vitamin B-2) and health. American Journal of Clinical Nutrition, 77(6), 1352-1360. doi: 10.1093/ajcn/77.6.1352
Sahlin, K., Sallstedt, E.K., Bishop, D., & Tonkonogi, M. (2008). Turning down lipid oxidation during heavy exercise—what is the mechanism? Journal of Physiological Pharmacology, 59, 19-30. Retrieved from https://www.ncbi.nlm.nih.gov/pubmed/19258655
Tong, L., & Harwood, H.J. (2006). Acetyl-coenzyme A carboxylases: Versatile targets for drug discovery. Journal of Cell Biochemistry, 15(99), 1476-1488. doi:10.1002/jcb.21077
Wutzke, K.D., & Lorenz, H. (2004). The effect of L-carnitine on fat oxidation, protein turnover, and body composition in slightly overweight subjects. Metabolism, 53(8), 1002-1006. Retrieved from https://www.sciencedirect.com/science/article/pii/S0026049504001647
Zhao, S., Li, R., Li, Y., Chen, W., Zhang, Y., & Chen, G. (2012). Roles of vitamin A status and retinoids in glucose and fatty acid metabolism. Biochem Cell Biology, 90(2), 142-152. doi: 10.1139/o11-079
Comentários